The search for genetic material has been a pivotal quest in understanding inheritance and the molecular basis of life itself. Through centuries of inquiry and experimentation, scientists have unraveled the role of DNA as the genetic material, elucidating the way it stores, replicates, and transmits genetic information from one generation to the next. This knowledge has laid the foundation for modern genetics, biotechnology, and medicine.
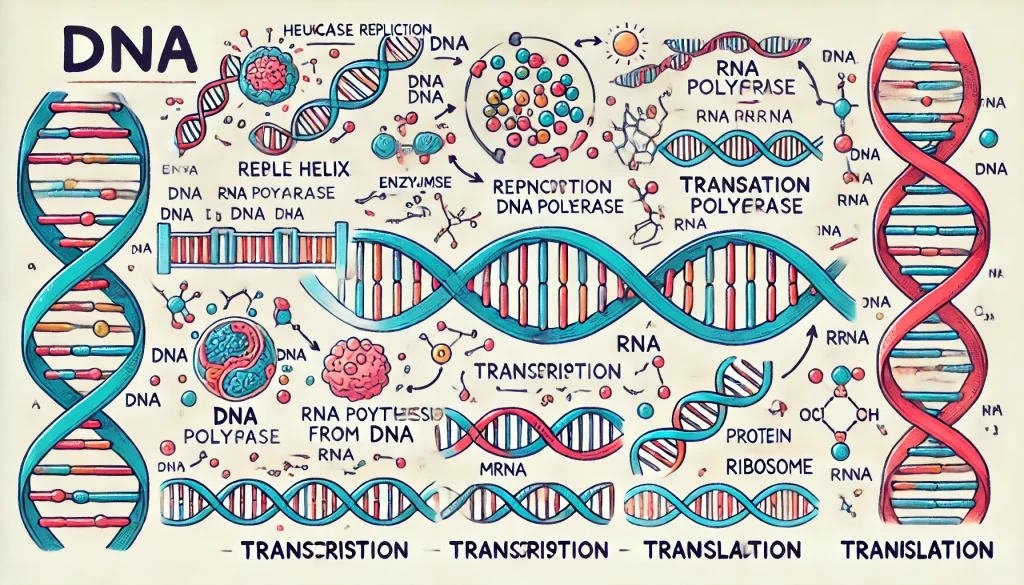
1. Historical Context: Early Theories of Heredity
The concept of inheritance has fascinated scientists since antiquity. Early scientists and philosophers speculated that traits were passed from parents to offspring, but the mechanisms were unknown. In the 19th century, Gregor Mendel’s experiments with pea plants provided the first scientific evidence for heredity patterns, identifying what he called “factors” (now known as genes) that determined specific traits. However, the biochemical nature of these hereditary factors remained unclear.
By the early 20th century, scientists recognized that chromosomes in the nucleus played a critical role in inheritance. The discovery of chromosomal structures led to the chromosome theory of inheritance, which suggested that chromosomes contained the genetic material. However, chromosomes consist of both DNA and proteins, and for decades, scientists debated whether DNA or proteins were the true carriers of genetic information. Proteins were initially favored due to their structural complexity and variety, which seemed necessary to encode the diversity of life.
2. Discovering DNA’s Role in Heredity
The search for the genetic material progressed significantly in the 20th century through a series of groundbreaking experiments. These key experiments include:
Frederick Griffith’s Transformation Experiment (1928)
In 1928, British bacteriologist Frederick Griffith conducted a transformative experiment while studying two strains of Streptococcus pneumoniae bacteria—one virulent (S strain) and one non-virulent (R strain). Griffith observed that when he killed the virulent S strain and mixed it with the living non-virulent R strain, the harmless R strain transformed into the virulent S strain, passing on virulence to its progeny. Griffith referred to this phenomenon as “transformation.” Although he did not identify the transforming substance, his work suggested the presence of a “transforming principle” that carried genetic information.
The Avery-MacLeod-McCarty Experiment (1944)
Building on Griffith’s findings, Oswald Avery, Colin MacLeod, and Maclyn McCarty sought to identify the transforming principle. They isolated different biomolecules from the S strain, including DNA, RNA, and proteins. They treated R strain bacteria with these purified substances and found that only DNA could transform the R strain into the virulent S strain. This experiment provided compelling evidence that DNA, not protein, was the genetic material responsible for carrying hereditary information. However, skepticism persisted in the scientific community, as some researchers continued to believe that proteins played a central role.
The Hershey-Chase Experiment (1952)
In 1952, Alfred Hershey and Martha Chase conducted a crucial experiment using bacteriophages (viruses that infect bacteria). These phages consisted of only DNA and protein, making them ideal for determining which molecule carried genetic material. Hershey and Chase labeled the DNA with radioactive phosphorus-32 and the protein coat with radioactive sulfur-35, allowing them to track each component. After the bacteriophages infected bacterial cells, only the radioactive phosphorus was found inside the cells, while the radioactive sulfur remained outside. This experiment confirmed that DNA, not protein, was the genetic material injected by the phage to direct bacterial replication, conclusively establishing DNA as the carrier of genetic information.
3. Understanding DNA Structure
Once DNA was identified as the genetic material, understanding its structure became essential for uncovering how it stored and transmitted genetic information.
Chargaff’s Rules
In the 1940s, biochemist Erwin Chargaff observed that in any given DNA molecule, the amount of adenine (A) was equal to the amount of thymine (T), and the amount of cytosine (C) equaled the amount of guanine (G). This observation, now known as Chargaff’s rules, hinted at a specific pairing mechanism within DNA but did not reveal the complete structure.
The Discovery of the Double Helix
In 1953, James Watson and Francis Crick proposed the double helix model of DNA structure, drawing on X-ray diffraction images produced by Rosalind Franklin and Maurice Wilkins. Their model demonstrated that DNA is composed of two strands twisted into a helical structure, held together by complementary base pairs (A with T, and C with G) connected by hydrogen bonds. The antiparallel orientation of the strands and the specific base-pairing rules explained Chargaff’s observations. The double helix model also suggested a potential mechanism for DNA replication, which became a cornerstone of molecular biology.
4. DNA Replication: Preserving Genetic Information
The molecular basis of inheritance relies on the faithful replication of DNA, ensuring that genetic information is accurately passed to the next generation. DNA replication occurs during cell division, enabling each daughter cell to receive an identical copy of the genetic material. This process involves:
Semiconservative Replication
Watson and Crick’s model suggested a “semiconservative” replication mechanism, which was later confirmed by the Meselson-Stahl experiment. In semiconservative replication, each of the two strands in the double helix serves as a template for synthesizing a new complementary strand. This results in two DNA molecules, each consisting of one original and one newly synthesized strand, preserving the sequence information.
Key Enzymes in DNA Replication
DNA replication involves various enzymes that work together in a precise sequence. Helicase unwinds the DNA double helix, creating a replication fork where replication occurs. DNA polymerase synthesizes new DNA strands by adding nucleotides complementary to the template strand, while DNA ligase seals fragments to ensure a continuous strand. These enzymes work with remarkable accuracy and fidelity, supported by proofreading mechanisms that correct replication errors.
5. Transcription and Translation: From DNA to Protein
The molecular basis of inheritance extends beyond DNA replication, encompassing how genetic information is expressed as functional proteins, which ultimately determine an organism’s traits. This process occurs in two stages:
Transcription
In transcription, DNA serves as a template to synthesize messenger RNA (mRNA). RNA polymerase binds to a specific DNA sequence called the promoter, initiating RNA synthesis. The mRNA strand is complementary to the DNA template strand, except that thymine (T) is replaced by uracil (U) in RNA. This mRNA molecule carries the genetic instructions from the DNA in the nucleus to the ribosomes, where proteins are synthesized.
Translation
In translation, the mRNA sequence is used to synthesize a protein. Ribosomes read the mRNA sequence in sets of three nucleotides, called codons, each corresponding to a specific amino acid. Transfer RNA (tRNA) molecules bring the appropriate amino acids to the ribosome, where they are joined together to form a polypeptide chain. The sequence of amino acids in the protein determines its structure and function, linking the genetic code in DNA to the physical traits of an organism.
6. Genetic Mutations: Variability and Inheritance
While DNA replication is highly accurate, occasional errors, or mutations, occur. Mutations can alter the genetic information and produce variations in organisms, contributing to evolution and diversity. Mutations can be classified into various types, such as point mutations (single nucleotide changes), insertions, deletions, and chromosomal rearrangements.
Some mutations are silent, having no effect on the phenotype, while others can lead to significant changes in protein structure and function. In some cases, mutations can be beneficial, conferring adaptive advantages that drive natural selection. In others, mutations can cause genetic disorders or predispose individuals to diseases, highlighting the importance of genetic stability for maintaining organismal health.
7. Epigenetics: Beyond the DNA Sequence
The molecular basis of inheritance is not limited to DNA sequence alone. Epigenetics refers to heritable changes in gene expression that do not involve alterations in the DNA sequence. Epigenetic mechanisms, such as DNA methylation and histone modification, regulate gene activity, influencing development, cell differentiation, and responses to environmental factors.
Epigenetic modifications can be passed down through cell divisions, affecting gene expression in offspring. These modifications are influenced by environmental factors, such as diet, stress, and exposure to toxins, demonstrating that inheritance is shaped by a complex interplay between genes and the environment.
8. Conclusion: The Impact of Understanding Genetic Material
The discovery of DNA as the genetic material and the elucidation of its structure, replication, and expression mechanisms have transformed biology and medicine. This understanding has led to groundbreaking advances in genetic engineering, biotechnology, and personalized medicine. Techniques such as DNA sequencing, gene editing (CRISPR-Cas9), and genetic testing have enabled scientists to manipulate genetic material, diagnose genetic disorders, and develop targeted therapies.
In summary, the search for genetic material has revealed DNA as the blueprint of life, encoding the instructions for biological diversity and inheritance. Through continued research, our understanding of the molecular basis of inheritance will deepen, paving the way for innovations that will further enhance human health, agriculture, and the environment.
10 Questions and Answers Molecular basis of inheritance:
1. What is DNA, and why is it called the “genetic material”?
- Answer: DNA (deoxyribonucleic acid) is a molecule that carries genetic information necessary for the growth, development, and reproduction of all living organisms. It is called the “genetic material” because it encodes the instructions for building and maintaining an organism and is passed from parents to offspring. The specific sequence of nucleotide bases (adenine, thymine, cytosine, and guanine) in DNA forms genes, which dictate various traits and biological functions.
2. How did Frederick Griffith’s experiment contribute to our understanding of genetic material?
- Answer: Griffith’s experiment in 1928 demonstrated a process he termed “transformation.” He discovered that non-virulent bacteria could acquire virulence by taking up genetic material from dead, virulent bacteria. This suggested that some “transforming principle” was responsible for transmitting hereditary traits, providing early evidence that genetic information could be passed between cells. Although he did not identify the substance, this experiment set the stage for later discoveries that DNA was the genetic material.
3. What was the Avery-MacLeod-McCarty experiment, and what did it show?
- Answer: The Avery-MacLeod-McCarty experiment, conducted in 1944, aimed to identify the “transforming principle” from Griffith’s experiment. By isolating and purifying DNA, RNA, and protein from virulent bacteria and exposing non-virulent bacteria to each, they found that only DNA could transform non-virulent bacteria into virulent ones. This experiment provided strong evidence that DNA, rather than protein, was the molecule responsible for heredity.
4. Why was the Hershey-Chase experiment significant in confirming DNA as the genetic material?
- Answer: The Hershey-Chase experiment in 1952 used bacteriophages (viruses that infect bacteria) to show that DNA is the genetic material. By labeling the DNA and protein of the virus with different radioactive markers, they observed that only DNA entered the bacterial cells during infection, while the protein did not. This provided conclusive proof that DNA, not protein, carries genetic information.
5. What are Chargaff’s rules, and why are they important?
- Answer: Chargaff’s rules state that in any DNA sample, the amount of adenine (A) equals thymine (T), and the amount of cytosine (C) equals guanine (G). This finding was important because it hinted at a complementary base-pairing mechanism in DNA, which later helped Watson and Crick discover the double helix structure. Chargaff’s rules are fundamental to understanding DNA’s structure and function.
6. How does the double-helix structure of DNA enable it to store genetic information?
- Answer: The double-helix structure of DNA allows it to store genetic information through a specific sequence of nitrogenous bases (A, T, C, G) along each strand. The complementary base-pairing (A with T and C with G) ensures that each strand can serve as a template for replication. This structure allows DNA to carry a vast amount of genetic information and pass it accurately to offspring, supporting inheritance and cellular function.
7. What is DNA replication, and why is it called “semiconservative”?
- Answer: DNA replication is the process by which DNA makes an identical copy of itself before cell division. It is called “semiconservative” because each new DNA molecule consists of one original (parent) strand and one newly synthesized strand. This method of replication helps preserve the genetic information across generations by maintaining the original sequence in each daughter cell.
8. What roles do enzymes like helicase and DNA polymerase play in DNA replication?
- Answer: Helicase is an enzyme that unwinds the double helix by breaking the hydrogen bonds between the DNA strands, creating a replication fork. DNA polymerase is an enzyme that synthesizes the new DNA strand by adding complementary nucleotides to the template strand. These enzymes ensure that DNA replication proceeds accurately and efficiently, allowing cells to divide with an intact set of genetic information.
9. What is transcription, and how does it relate to gene expression?
- Answer: Transcription is the process of synthesizing messenger RNA (mRNA) from a DNA template. During transcription, RNA polymerase binds to a gene’s promoter and produces an mRNA strand that is complementary to the DNA template. This mRNA then travels to the ribosome, where it guides protein synthesis. Transcription is the first step in gene expression, translating genetic instructions from DNA into functional proteins that define an organism’s traits.
10. What is the significance of epigenetics in inheritance?
- Answer: Epigenetics involves changes in gene expression that do not alter the DNA sequence itself but can still be passed on to future generations. Epigenetic modifications, like DNA methylation and histone modification, regulate gene activity and can be influenced by environmental factors (such as diet or stress). Epigenetics adds an additional layer of complexity to inheritance, showing that traits can be influenced by both genetic and environmental factors without changing the DNA sequence itself.